With JWST, a first glimpse of stars in the neighborhood of massive black holes in the early universe
For the first time, astronomers have observed starlight from a galaxy that hosts a quasar (an active supermassive black hole) in the early universe, less than one billion years after the Big Bang. The group, which includes astronomers from the Max Planck Institute for Astronomy, used the James Webb Space Telescope (JWST) for their observations. For the two quasar-galaxy systems observed, the researchers were able to determine both the stellar mass of the galaxy and the mass of the black hole powering the quasar. The masses are related in the same way as for the present-day galaxies we see around us – a fact that presents a challenge for models of the evolution of galaxies and their black holes.
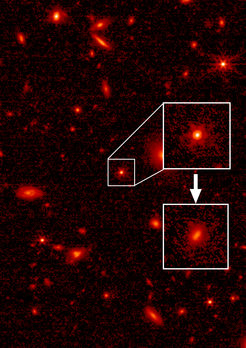
Quasars are some of the most extreme objects in the universe – they are among the brightest objects out there, outshining whole galaxies, and behind all that brightness is some of the most extreme physics we know: Quasar light is produced as gas falls into black holes at the centers of galaxies, and such supermassive black holes embody the mass of millions or even billions of Suns. Now a group of astronomers led by Masafusa Onoue, started when Onoue was a postdoctoral researcher at the Max Planck Institute for Astronomy, and using the James Webb Space Telescope, have managed for the first time to observe the host galaxies of quasars in the distant cosmic past, at a time when the universe was less than 10% of its current age.
The observations show that, in one important respect, those early quasars and their host galaxies are very similar to modern galaxies: For modern galaxies, there is a direct correlation between the mass of a galaxy’s supermassive black hole and the total mass of the galaxy’s stars. The early quasars the astronomers observed show the same relation. It is not, at this point, clear what this means and how this comes about – but it is likely to be relevant for attempts to solve a persistent riddle about early supermassive black holes, namely how they managed to accumulate a comparatively large amount of mass in a comparatively short period of cosmic time.
Supermassive black holes and a curious relation
Since the discovery of the first quasar in 1963, astronomers have learned an enormous amount about these objects, from the mechanisms producing their intense radiation to their influence on their surrounding galaxies. A key insight was that quasars are just the tip of the iceberg – every larger galaxy has a central, supermassive black hole, but most of them are not nearly as ostentatious as their quasar brethren (If you are following astronomy news, you will have seen the “image” taken of the shadow of our own galaxy’s central black hole that were published in 2019.)
Since the early 2000s, it emerged that there is a direct relation between the mass of a galaxy’s central black hole and the total mass of the galaxy’s stars. If you plot these masses for different galaxies, there is a comparatively simple function (a power law) linking the two. Given that stellar masses of galaxies are so much larger – by about a factor of 1000 – than central black hole masses, that relationship, which holds for million-solar mass central black holes as well as it does for specimens that are 100,000 times more massive, is remarkable.
Black holes and their galaxies: a common history
A fairly obvious assumption is that this relation between black holes and their galaxies has come about because of these object’s common history – that somehow, the same scenario that will lead to the formation of a galaxy with high stellar mass will also lead to the evolution of a more massive central black hole, and vice versa. One proposal was recently worked out quantitatively by Knud Jahnke (an MPIA scientist involved in the research reported on here) and his colleague Andrea Macciò. That proposal emphasizes the role of averaging effects: In the current cosmological scenarios, galaxies as a whole grow through the merger with and incorporation of smaller galaxies, and central black holes through the merger with and incorporation of those smaller galaxies’ central black holes. The natural consequence after numerous mergers is that the galaxy mass will tend to the average mass of the initial galaxy population times the number of galaxies that were incorporated, while the central black hole mass will tend to the average mass of initial black holes, times the same number, leading to a roughly linear relationship.
Other scenarios have explored causal relationships between black hole and galaxy mass. Gas falling into the central black hole would transform the black hole into a quasar. The quasar’s radiation could in turn influence the reservoir of gas in the galaxy, which provides the raw material for both black hole growth and star formation in the galaxy. If that gas is heated up by the quasar, this would at the same time hamper star formation and throttle the flow of gas to the central black hole. Self-regulation cycles of this kind have been proposed, linking star formation on the scales of a whole galaxy with black hole growth in galaxies’ centers.
A bonus riddle: fast black hole growth
There is an additional riddle linked to the evolution of supermassive black holes. Quasars, being as bright as they are, can be studied out to enormous distances. In astronomy, observing distant objects always means that one is observing the distant past: It takes time for these object’s light to reach us, which means that we see those objects as they were long ago, when the light now reaching our telescopes was emitted. It takes light of the most distant quasars more than 13 billion years to reach us, meaning that we see those quasars as they were more than 13 billion years ago – some hundred million years after our universe’s initial hot Big Bang phase.
The supermassive black holes powering those most distant known quasars have surprisingly high masses – surprising because those seven or eight hundred million years since the Big Bang were not, cosmically speaking, a lot of time to gather the billions of solar masses those early quasars appear to have. While a number of scenarios have been proposed, the issue of how those early supermassive black holes managed to grow as fast as they did is not, at this time, settled.
Observing quasar host galaxies
While a quasar is comparatively easy to observe, the surrounding galaxy is not. Since a quasar typically is more than five times brighter than all the stars of its host galaxy taken together, it tends to outshine its host galaxy. Separating quasar and galaxy is a job for space telescopes – even for the best ground-based telescopes, the detrimental influence of Earth’s atmosphere makes it difficult to cleanly distinguish the light from the quasar (which, for an observer on or near Earth, looks point-like) from the light of the surrounding host galaxy. For quasars whose light takes up to about 10 billion years to reach us, the Hubble Space Telescope was highly successful in imaging quasar host galaxies.
But for more distant quasars, Hubble runs into difficulties. The first of two main reasons is cosmic expansion – the fact that, over the course of cosmic history, the large-scale distances between galaxies increase. This leads to what is called the cosmological redshifts: light from distant galaxies is stretched to ever longer wavelengths (“redshifted”). For host galaxies whose light takes more than about 10 billion years to reach us, starlight is firmly shifted into the infrared part of the electromagnetic spectrum. The second is Hubble’s low orbit close to Earth, with its continuously changing conditions of temperature and light, that ever so slightly limit Hubble’s ability to sufficiently characterize the light from the (point-like) quasars.
JWST and quasar host galaxies
This is where JWST comes into its own: JWST, operated by NASA, ESA, and the Canadian Space Agency CSA, was launched in late 2021. As an infrared space telescope, placed 1.5 million kilometers from Earth, JWST is perfectly equipped to observe those very distant quasars and their host galaxies. That is why the team led by Masafusa Onoue, then at MPIA, proposed to observe 12 of the lowest luminosity quasars known in the early Universe in the first operational year of JWST. As quasars go, these are not particularly bright, increasing the chances of observing the host galaxies. The first two quasars that were actually observed by JWST, called J2236+0032 and J2255+0251 (which encodes the object’s position in the sky), were discovered in 2015 and 2017 using a ground-based telescope, the Subaru telescope operated by the National Observatory of Japan on Mauna Kea on Hawai’i. Light from these quasars has taken 12.9 and 12.8 billion years to reach us, respectively, showing us the two quasars as they were 870 and 880 million years after the Big Bang.
In October and November 2022, the astronomers used the JWST – which at the time had been in operation for only a few months – to observe each of the quasars for almost 2 hours at two different wavelengths, using the NIRCam near-infrared camera. Knud Jahnke says: “25 years ago, it was amazing to us that we were able to observe host galaxies from 3 billion years back, using large ground-based telescopes. The Hubble Space Telescope allowed us to probe the peak epoch of black hole growth 10 billion years ago. And now we have JWST available to see the galaxies in which the first supermassive black holes emerged.”
The host galaxy became visible after the researchers had carefully modelled and then subtracted the compact central light of the quasar, revealing the most distant starlight from a quasar host galaxy yet observed. Going by the amount of starlight, the galaxies weigh in at 130 and 30 billion solar masses, respectively – for comparison: the stellar mass of our home galaxy, the Milky Way, amounts to about 60 billion solar masses.
Black hole masses and a familiar relation
The astronomers also used JWST’s near-infrared spectrograph NIRSpec to analyse the combined spectrum of the quasar and the host galaxy. Certain spectral features, the so-called “broad lines,” are commonly assumed to be due to gas that is in close orbit around a quasar’s black hole. The properties of those features are directly related to the speed at which the gas orbits, which in turn is related to the black hole mass. By this route, the researchers estimated the black hole masses of the two quasars at 1.4 billion and 200 million solar masses, respectively. (Our home galaxy’s black hole is only about 4 million solar masses.)
When the astronomers added their two early-universe data points to the existing relations of the local present universe, they fit the pattern surprisingly well. Even though what they saw was a snapshot from the early universe, less than 1 billion years after the Big Bang, the galaxies and their black holes seemed to obey the same relationship of black hole mass and total stellar mass as their modern-day counterparts.
So what does it mean?
Science usually is not a matter of heureka moments, and this new data is no exception. The new findings are clearly important for the efforts to understand both the co-evolution of galaxies and their central black holes, and the efforts to understand rapid black hole growth in the early universe. But given the current state of the field, the data alone is not enough to favour, or rule out, specific models. It will doubtlessly play an important role as future, more complete scenarios for the interplay between galaxies and their black holes are formulated.
By that time, there should be significantly more data. The proposal on which the present results for two quasars are based included a total of 12 quasars and 50 hours of JWST observation time. About half of the additional not-that-luminous quasars have already been observed, and the data are being analysed. In addition, the astronomers were awarded another 11 hours of observation time in the 2023/2024 season of JWST observations (“Cycle 2”), to revisit one of the present two quasars and its host galaxy.
Background information
The research described here has been published as Xuheng Ding et al., “Detection of stellar light from quasar host galaxies at redshifts above 6” in the journal Nature.
The MPIA scientists involved are Masafusa Onoue (overall project lead, at MPIA from 2018 to 2021, now Kavli Institute for Astronomy and Astrophysics, Peking University, China and Kavli Institute for the Physics and Mathematics of the Universe [Kavli IPMU], and the University of Tokyo, Japan), Knud Jahnke, Melanie Habouzit, and Fabian Walter
in collaboration with
Xuheng Ding (paper lead, Kavli IPMU) and John D. Silverman (Kavli IPMU), and other scientists of the SHELLQs survey and project."